Numerical Modeling of Mining Sequences and Caving
The Kiirunavaara Mine is a large-scale sublevel caving (SLC) mine located near the city of Kiruna in northern Sweden and owned and operated by LKAB (Luossavaara-Kiirunavaara AB). The mine has an annual production of about 28 Mt, with the deepest extraction point at about 1000 m depth from ground surface. A large magnitude seismic event (4.2 Mw) in 2020 caused significant damage to the mine infrastructure over a large volume, leading to the disruption of mining activities in heavily damaged areas. Several reopening mining sequences were analyzed in FLAC3D for the resumption of production in these areas.
Project Background
The Kiirunavaara Mine is a deep SLC mine in northern Sweden. Like many other hard rock mines mining at depth, mining-induced seismic events are a concern, which rise in severity as mining progresses. A large seismic event (4.2 Mw) occurred in 2020 in production block 22, leading to extensive damage to the mining infrastructure affecting the overall mine output for months.
Because of the significant damage to infrastructure, it was not possible to resume mining directly in the event area. Mining is instead resumed from lower levels by creating a remnant pillar between the existing sublevel and the resumed mining. Itasca conducted three-dimensional numerical analyses using FLAC3D to study the behavior of the pillar and assess the rock mechanical aspects of the alternative sequences. Coupling of FLAC3D and CAVESIM was used to simulate material flow and cave propagation.
FLAC3D Geomechanical Models
To accurately simulate the 3D stress field in the damaged area, a mine-scale (global) model was created. The global model included all major geological formations in the area as well as the mined stopes, caved rock, and large-scale geological structures. The ground surface topography was duplicated from a digital terrain model with some simplifications, as shown below.
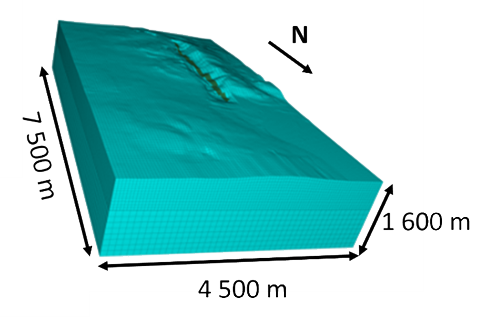
To explicitly investigate the pillar behavior in production block 22, a smaller scale (local) model was also created, including details in local geology and production sequencing. The local model was built as a hybrid mesh combining a FLAC3D generated oct-tree mesh with a tetrahedral mesh built in the CAD-software Rhino along with the Itasca pre-processor Griddle.
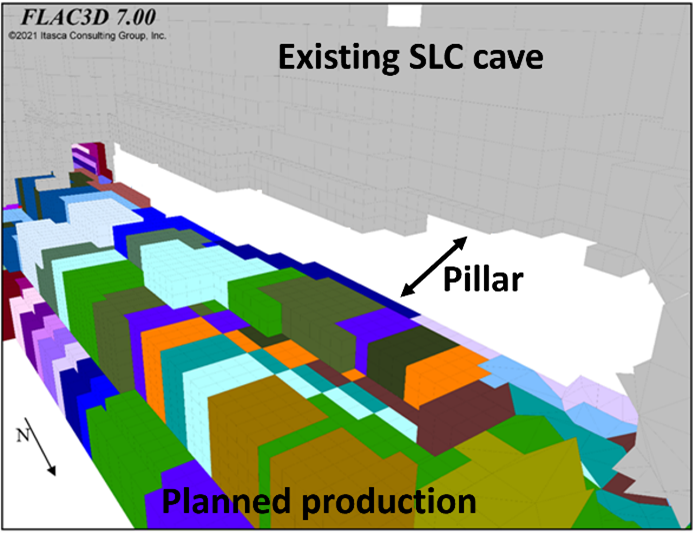
Constitutive Model
Both the global and local FLAC3D models used the Itasca constitutive model for Advanced Strain Softening (IMASS) for all geological entities. Strength weakening in the IMASS constitutive model is defined by three Hoek-Brown strength envelopes: a peak envelope and two residual strength envelopes.
The peak behavior is based on the built-in modified Hoek-Brown model. The peak strength envelope parameters are calculated from the classic Hoek-Brown equations based on GSI and intact rock parameters UCS and mi.
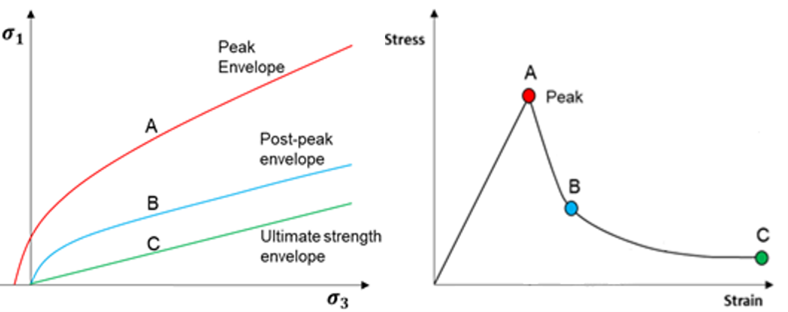
The two residual strength envelopes describe the rock mass softening during plastic straining. At the first residual strength envelope (post-peak), the rock mass is assumed to have undergone fracturing, but the resulting rock fragments are still fully interlocked. The second residual strength envelope (ultimate strength) represents the bulked rock mass residual strength with a minimum degree of interlocking. The strain region between the first and second residual envelopes describes the behavior of a cohesionless, perfectly frictional material with progressively decreasing interlocking, allowing a realistic strain-softening behavior to be modeled.
Sloss (a damage indicator in IMASS)
In this project, the "sloss" indicator of damage was used to evaluate the amount of softening/ weakening that the rock mass had undergone. Sloss varies between 1 and -1.
Sloss is schematically represented below for different degrees of damage and disturbance in a rock mass. At “A” and “B” the rock mass is assumed to be predominantly intact. At “C” the rock mass has undergone fracturing, but the resulting rock fragments are still fully interlocked, while at “D” the degree of rock fragments interlocking is at its minimum and the porosity is maximized. A calibrated sloss to field observations in terms of damage can be a powerful tool for prediction of the serviceability and stability of the structure.
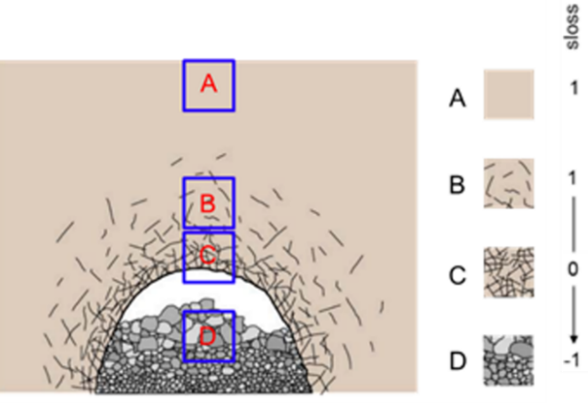
Stress State
A shared coordinate system between the local and global models was used, enabling the calculated stress state from the global model to be superimposed directly onto the local model by the retrieval of the stress state for each zone centroid position, as shown below.
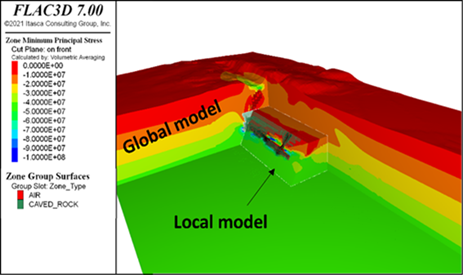
Material Flow and Cave Propagation
For the local model, the code coupling between FLAC3D and CAVESIM was used to model the caving propagation. Material draw in CAVESIM is modeled on a ring-by-ring basis. The draw column from each ring interacts with the draw from adjacent rings and previously mobilized material, allowing emergent draw patterns to form inside the cave rock column, which are relayed to FLAC3D as flow rates. The flow rates are then used by FLAC3D to calculate the back pressure from the cave and subsequently the stress redistribution caused by the discontinuous flow.
The combined information from FLAC3D and CAVESIM allows for emergent caving to form based on the draw pattern and the geomechanical properties of the host and rock, including air gaps from delayed caving. The emergent cave in turn affects the deformation and stress redistribution patterns around the cave column based on the differential flow rates inside the cave, with flowing regions supplying less confinement to the cave boundary than stagnated regions.
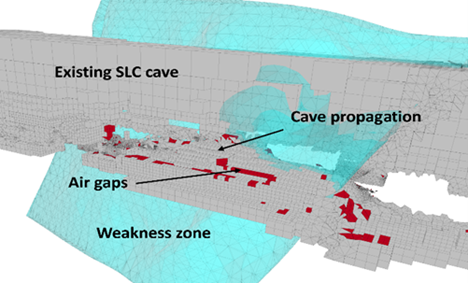
Resumption of Production
The results of the analyses performed have aided in the selection of a suitable mining plan to reopen and resume production in the damaged area. From the modeling effort it was concluded that confinement of the remnant pillar was the major factor controlling the pillar caving and seismic potential. To alleviate the seismic hazard from an uncontrolled pillar collapse, a mining sequence that could facilitate a controlled pillar caving was chosen as the best option for reopening the closed areas.